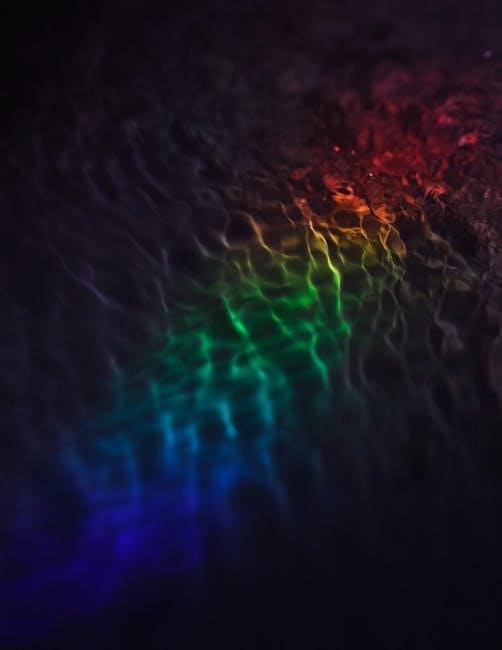
Mass spectrometry (MS) is a powerful analytical technique used to identify and quantify compounds by ionizing them and measuring their mass-to-charge ratio. It provides detailed insights into molecular structures, is highly sensitive, and has applications in proteomics, environmental science, and pharmaceutical analysis, making it indispensable across scientific fields.
1.1 What is Mass Spectrometry?
Mass spectrometry (MS) is a powerful analytical technique that identifies and quantifies compounds by ionizing them and measuring their mass-to-charge ratio (m/z). It involves converting molecules into gas-phase ions, which are then separated and detected based on their m/z values. This method provides detailed insights into molecular structures, making it highly versatile for applications in chemistry, biology, and environmental science.
1.2 Importance of Mass Spectrometry in Analytical Science
Mass spectrometry is a cornerstone in analytical science, offering high sensitivity and versatility for identifying and quantifying compounds. Its ability to provide detailed molecular information makes it indispensable in environmental monitoring, pharmaceutical analysis, and proteomics. By enabling precise detection of trace substances, MS plays a critical role in advancing research and ensuring quality control across diverse scientific fields, making it an essential tool for modern analytical challenges.
Understanding the Mass Spectrum
A mass spectrum plots ion signal intensity against mass-to-charge ratio, displaying peaks that represent ions’ relative abundance. It provides insights into molecular composition and structure.
2.1 Definition and Representation of a Mass Spectrum
A mass spectrum is a graphical representation of ion intensity plotted against the mass-to-charge ratio (m/z). It consists of peaks, each corresponding to specific ions, with their heights indicating relative abundance. The spectrum is a two-dimensional plot where the x-axis shows m/z values, and the y-axis represents signal intensity, providing a fingerprint for molecular identification and analysis.
2.2 Key Components of a Mass Spectrum
A mass spectrum contains several key components, including the molecular ion peak, base peak, isotopic peaks, and fragment ions. The molecular ion peak represents the intact molecule’s mass, while the base peak is the most intense. Isotopic peaks provide information about the molecular formula, and fragment ions reveal structural details. These elements collectively form the spectrum’s unique fingerprint, enabling compound identification and analysis.
Mass Spectrometry Instrumentation
Mass spectrometry instrumentation includes ionization sources, mass analyzers, and detectors. Techniques like MALDI-TOF and GC-MS combine ionization methods with analyzers to produce precise mass spectra for analysis.
3.1 Ionization Techniques in Mass Spectrometry
Common ionization techniques in mass spectrometry include MALDI (Matrix-Assisted Laser Desorption/Ionization) and ESI (Electrospray Ionization). MALDI uses a laser to ionize large molecules, while ESI ionizes samples in solution. EI (Electron Ionization) is often used for small molecules, producing stable ions for analysis. These techniques ensure effective ion formation, enabling accurate mass spectrum generation for compound identification and structural analysis.
3;2 Types of Mass Analyzers
Mass analyzers are critical components of mass spectrometers, separating ions by their mass-to-charge ratio. Common types include Quadrupole, Time-of-Flight (ToF), Orbitrap, and Ion Trap analyzers. Quadrupole analyzers are widely used for their simplicity and versatility in routine analysis. Time-of-Flight analyzers offer high mass accuracy and resolution, making them ideal for complex proteomics studies. Orbitrap analyzers provide exceptional mass accuracy for detailed structural analysis, while Ion Trap analyzers enable tandem MS and are highly versatile for quantitative studies.
Fragmentation Patterns in Mass Spectrometry
Fragmentation patterns reveal how molecules break apart, producing characteristic ions. Common types include cleavage of bonds and rearrangements, influenced by ionization methods and molecular stability.
4.1 Common Fragmentation Types
Common fragmentation types include beta cleavage, McLafferty rearrangement, and alpha cleavage. Beta cleavage involves breaking bonds adjacent to a functional group, often in carbonyl-containing compounds. The McLafferty rearrangement occurs in molecules with a gamma-hydrogen, leading to a characteristic peak. Alpha cleavage involves breaking bonds adjacent to a charged site, typically in smaller molecules. These patterns help identify structural features and functional groups, aiding in compound identification and molecular formula determination. Fragmentation types vary based on the stability of the resulting ions and the energy applied during ionization, making them essential for interpreting mass spectra accurately.
4.2 Factors Influencing Fragmentation
Fragmentation in mass spectrometry is influenced by ionization methods, energy levels, and molecular structure. High-energy ionization techniques, like electron ionization, promote extensive fragmentation, while soft ionization methods, such as electrospray, preserve larger molecules. The stability of ions and the presence of functional groups also affect fragmentation patterns. Additionally, the sample’s preparation and the mass spectrometer’s settings can alter fragmentation outcomes, making it crucial to optimize conditions for accurate compound identification and structural analysis.
Applications of Mass Spectrometry
Mass spectrometry is widely applied in proteomics, environmental monitoring, food safety, and forensic analysis. It aids in disease diagnosis, drug development, and tracing biomarkers, enhancing research and industrial processes.
5.1 Proteomics and Metabolomics
Mass spectrometry is integral to proteomics, enabling protein identification, quantification, and structural analysis. It aids in studying post-translational modifications and protein-protein interactions. In metabolomics, MS identifies and quantifies metabolites, mapping metabolic pathways. Tandem MS and imaging techniques reveal spatial distributions of proteins and metabolites in tissues, advancing disease research, such as cancer and neurological disorders, by uncovering biomarkers and metabolic alterations.
5.2 Environmental and Pharmaceutical Analysis
Mass spectrometry is crucial in environmental monitoring for detecting pollutants and toxins in water and air. In pharmaceuticals, it aids in drug discovery, quality control, and metabolite identification. MS ensures compliance with regulatory standards by analyzing drug impurities and degradation products. Its high sensitivity and accuracy make it essential for trace analysis in both fields, ensuring safety and efficacy in pharmaceutical products and environmental sustainability.
Interpreting Mass Spectra
Interpreting mass spectra involves identifying molecular ions, base peaks, and fragmentation patterns to deduce molecular formulas and structural information, with IR data aiding compound identification.
6.1 Steps for Spectrum Interpretation
Interpreting a mass spectrum involves identifying the molecular ion peak, base peak, and significant fragments. Calculate the molecular formula by determining the mass-to-charge ratio. Analyze fragmentation patterns to deduce structural information. Use IR data to confirm functional groups. Identify common losses and rearrangements, such as McLafferty rearrangements. Compare with reference spectra for compound identification. Accurate interpretation requires practice and understanding of ionization techniques and fragmentation mechanisms.
6.2 Using IR Data for Compound Identification
IR spectroscopy complements mass spectrometry by providing functional group information. Key absorption bands in IR spectra help identify molecular structures, such as carbonyl groups (C=O) or hydroxyl groups (O-H). By correlating IR data with mass spectral fragmentation patterns, analysts can confirm molecular identities. This combined approach enhances accuracy in compound identification, especially for complex or unknown samples, and is widely used in organic and pharmaceutical analysis. It streamlines structural elucidation processes effectively.
Challenges in Mass Spectrometry
Key challenges include complex instrumentation, high costs, and interpreting large datasets. Sample preparation and ionization efficiency also pose difficulties, requiring expertise for accurate and reliable results.
7.1 Limitations of Mass Spectrometry Techniques
Mass spectrometry faces limitations such as high instrument costs, complex data interpretation, and challenges in ionizing large or fragile molecules. Sample preparation is critical, and matrix effects can affect accuracy. Additionally, isobaric interferences and limited dynamic range pose challenges in quantitative analysis. Expertise is required for optimal operation and data processing, making it less accessible for routine applications without specialized training.
7.2 Overcoming Instrumentation and Data Interpretation Challenges
Advancements in mass spectrometry address instrumentation and data interpretation challenges through improved mass analyzers and user-friendly software. High-resolution systems enhance accuracy, while automated data processing tools simplify analysis. Training and resources help researchers master complex techniques, enabling better decision-making and application across diverse fields, ensuring the technique remains a cornerstone of modern analytical science with continuous innovation to meet emerging demands.
Advances in Mass Spectrometry
Advances include tandem mass spectrometry (MS/MS) for precise molecular analysis and mass spectrometry imaging, enabling spatial distribution studies of molecules in tissues, enhancing sensitivity and application scope.
8.1 Tandem Mass Spectrometry (MS/MS)
Tandem mass spectrometry (MS/MS) involves multiple stages of mass filtering and fragmentation, enabling precise molecular analysis. It enhances sensitivity and specificity by isolating ions, fragmenting them, and analyzing the resulting fragments. This technique is particularly useful in proteomics and metabolomics for identifying complex biological molecules. MS/MS improves accuracy in compound identification and quantification, making it a cornerstone in advanced analytical workflows across scientific research and diagnostics.
8.2 Mass Spectrometry Imaging and Its Applications
Mass spectrometry imaging (MSI) spatially maps molecular distributions on tissue surfaces, offering insights into biological processes. By acquiring mass spectra across sample slices, MSI reveals protein, peptide, and metabolite localization. This technique aids in understanding disease mechanisms and drug distribution. Applications include pathology, pharmacology, and neuroscience, providing visualized molecular data crucial for research and diagnostics.
Resources for Learning Mass Spectrometry
Essential resources include books like “Fundamental Aspects of Organic Mass Spectrometry” and online databases like MetaboLights, offering tutorials and spectral data for comprehensive learning and research.
9.1 Recommended Books and Manuals
Key resources include “Fundamental Aspects of Organic Mass Spectrometry” and “A Beginner’s Guide to Mass Spectral Interpretation.” Manuals by experts like Karen Jonscher provide step-by-step guidance. “Mass Spectrometry: Principles and Applications” offers comprehensive insights, while “The Mass Spectrometry Handbook” covers advanced techniques. These texts are invaluable for understanding instrumentation, interpretation, and practical applications, catering to both novices and experienced professionals in the field.
9.2 Online Databases and Tutorials
Online resources like metabolic profiling tutorials by NV Breneva and protein spectrum databases by VN Penev are invaluable. Platforms offering mass spectrometry imaging guides and tandem MS/MS tutorials provide practical insights. Websites with downloadable PDFs, such as those detailing fragmentation patterns and IR data integration, enhance learning. These resources cater to both beginners and advanced users, offering step-by-step guidance and real-world applications in fields like proteomics and environmental analysis.
Future Trends in Mass Spectrometry
Future trends include advanced mass spectrometry imaging, high-resolution techniques, and portable devices. Integration of AI for data analysis and expanded applications in metabolomics and spatial biology are anticipated.
10.1 Emerging Technologies in Mass Spectrometry
Emerging technologies in mass spectrometry include advanced ion mobility systems, high-resolution mass analyzers, and portable mass spectrometers. Innovations like AI-driven data analysis and real-time sampling are enhancing sensitivity and speed. Mass spectrometry imaging is also evolving, enabling spatial mapping of molecules in tissues. These advancements promise to revolutionize fields like proteomics, metabolomics, and clinical diagnostics, offering unprecedented insights into complex biological systems and environmental monitoring.
10.2 Expanding Applications Across Scientific Fields
Mass spectrometry is revolutionizing diverse fields, from proteomics and metabolomics to environmental monitoring and pharmaceutical drug development. Its applications in food safety, forensic analysis, and medical diagnostics are growing rapidly. Advanced techniques enable precise identification of biomarkers, toxins, and contaminants. The ability to analyze complex biological samples and spatially map molecules in tissues is driving breakthroughs in medical research, ensuring MS remains a cornerstone of modern scientific investigation and innovation.